Vapor Intrusion
Site Investigation Tools
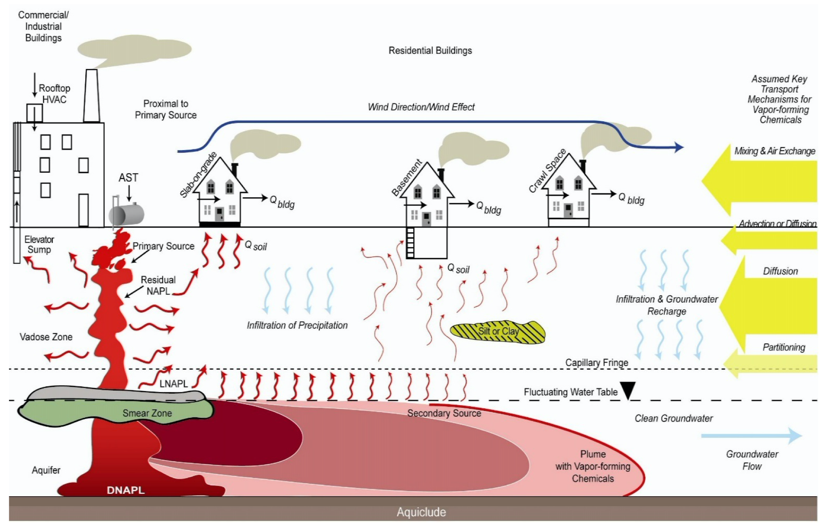
Figure 1. Example conceptual Site Model (EPA, 2015).
There are many tools available to environmental practitioners for investigating a site for vapor intrusion. As with any site investigation, an important early step is to develop a conceptual site model (CSM)1. One critical element of the CSM is an understanding of the type of volatile chemical that has been released into the subsurface. Some types of chemicals, such as petroleum hydrocarbons, degrade rapidly under aerobic conditions in the vadose zone, limiting the potential for vapor intrusion. Thus, the type of chemical present should inform the investigation strategy (ITRC, 2014 and EPA, 2015).
EPA's 2015 Vapor Intrusion Technical Guide recommends that the planning and data review team develop an initial CSM for vapor intrusion when the preliminary analysis indicates the presence of subsurface contamination with vapor-forming chemicals underlying or near buildings (Figure 1). This CSM is used to guide planning and scoping of the investigation and is updated and refined as additional information and insights are generated.
The vapor intrusion pathway is generally assessed by collecting, weighing, and evaluating multiple lines of evidence (e.g., hydrogeologic information in addition to soil, groundwater or vapor sampling data). Predictive modeling can be used to develop not only the CSM but also to plan appropriate sampling. Building design should be evaluated to determine how it affects the potential for vapor intrusion and vapor intrusion pathways. Click on the following sections for summaries of different lines of evidence for vapor intrusion and resources for further information:
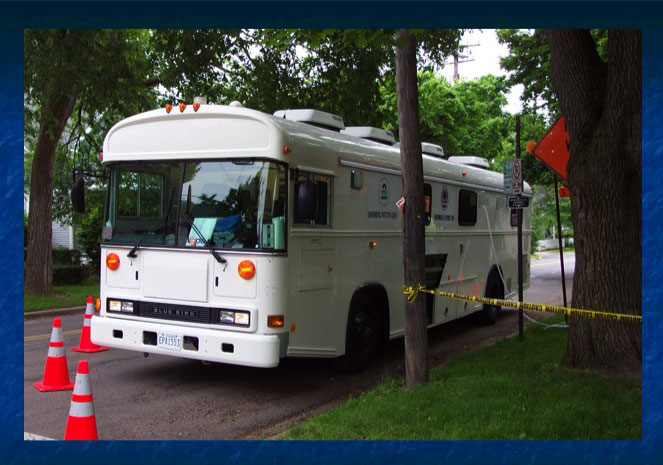
Figure 2. Trace Atomospheric Gas Analyzer (TAGA) Mobile Laboratory (U.S. EPA Region 5, 2020).
Sample collection for chemical analysis is the primary way in which a CSM is augmented and refined with site-specific data. Sampling may be needed to refine the understanding of the source and extent of contamination, as well as possible receptors and risk levels. The use of real-time analysis can expedite the investigation of vapor intrusion at a site. Figure 2 shows EPA's Trace Atmospheric Gas Analyzer (TAGA) mobile lab deployed in the field. Other commercial available portable gas chromatographs/mass spectrometers also provide the same capabilities (EPA Region V, 2020). Sampling may also help evaluate the amount of contamination present beneath the floor or foundation or inside a particular building. Table G-5 of ITRC's 2014 Petroleum Vapor Intrusion guide provides a matrix of measurement approaches and their appropriateness for evaluating vapor intrusion in different circumstances. The investigation toolbox in Appendix G was developed for both petroleum and non-petroleum vapor intrusion assessments. Table G-6 evaluates the advantages/disadvantages of investigative strategies. The strategies discussed here include:
- Groundwater Sampling
- Soil Gas Sampling
- Bulk Soil Sampling
- Sub-Slab Sampling
- Conduit/Utilities Sampling
- Air Sampling
- Analytical Methods
Groundwater Sampling
Contaminated groundwater may serve as a source for vapor intrusion into commercial and residential buildings. It is important to identify which groundwater contaminants are most likely to partition into a vapor phase that could potentially migrate into overlying structures. Section 6.3.1 of the EPA's 2015 Vapor Intrusion Technical Guide contains recommendations for characterizing groundwater plumes so that representative vapor source concentrations can be determined. Characterizing water table concentrations is key. To that end, the EPA recommends that groundwater samples be obtained from wells with short, screened intervals that span the water table. For additional information on various groundwater sampling methods and their associated pros and cons, see the Interstate Technology & Regulatory Council (ITRC) 's 2014 Petroleum Vapor Intrusion: Fundamentals of Screening, Investigation and Mitigation, Table G-1 Groundwater Sampling Methods for Vapor Intrusion Investigations.
↩Soil Gas Sampling
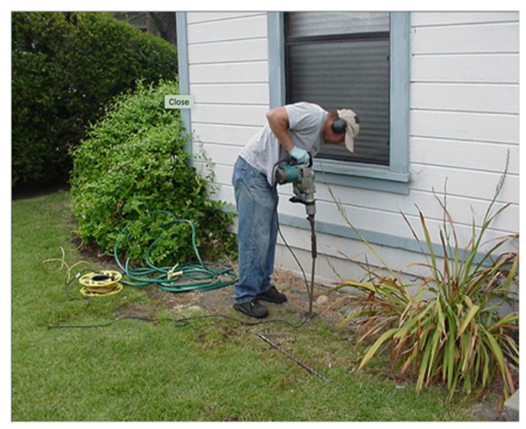
Figure 3. Advancing a Soil Gas Probe (U.S. EPA Region 5, 2020).
Soil gas samples are collected in the area of concern to determine the nature and extent of vapor contamination in the vadose zone. Figure 3 shows a soil gas probe being used to collect data near the foundation of a structure. Soil gas data can also help in the design and performance monitoring of soil vapor extraction systems. Soil gas surveys can employ either active2 or passive3 soil gas sampling techniques. Soil gas sampling methods are available from a variety of sources (e.g., EPA Region 5, 2020, ITRC 2014, and state-specific guidance). If vapor contamination is present, further characterization of nearby buildings might be warranted using approaches such as sub-slab soil gas and indoor air sampling.
↩Bulk Soil Sampling
Bulk soil sampling is useful in delineating source areas of VOC contamination (nature and extent) and determining the gross mass of contamination present in a source area. However, it is problematic to use bulk soil sampling to assess the potential for vapor intrusion exposure from VOCs in undisturbed soil or residual soil contamination left in an excavation following a removal action. As described in EPA's Challenges in Bulk Soil Sampling and Analysis for Vapor Intrusion Screening of Soil, (2014), the challenges in using bulk soil samples for vapor intrusion exposure calculations include:
- Volatilization and degradation losses - Significant losses of VOCs can occur during collection of the bulk samples. Samples must be prepared and preserved quickly in the field to limit losses of VOCs. Preservatives, such as methanol, used to prevent volatilization and degradation of the sample are flammable and can be dangerous to transport. A non-field-preservation method uses sampling devices that minimize VOC loss by containing the soil sample in a sealed zero headspace chamber. The sample can be stored up to 48 hours before analysis.
- Sensitivity of analytical methods - The method detection limits (MDLs) for bulk soil analytical methods when methanol is used as a preservative are typically higher than the calculated soil screening levels for vapor intrusion. In addition, for some VOCs such as trichloroethylene (TCE) and tetrachloroethylene (PCE), which are common vapor intrusion concerns, the non-methanol preservative method still has MDLs above the screening levels for vapor intrusion.
- Heterogeneity of soil and contaminant distribution - Soil properties such as fraction of organic carbon, porosity and moisture content can exhibit significant heterogeneities (i.e., vary considerably both laterally and with depth at a site). The scale of the heterogeneities may also vary. Additionally, soil moisture may exhibit temporal variability. These variabilities pose challenges when trying to use VOC vapor concentrations from discrete soil samples to estimate large-scale average VOC concentrations.
Bulk sampling is useful for determining the nature and extent of contamination. Field headspace techniques can be employed on bulk soil samples to rapidly determine the presence and concentrations of VOCs with enough precision to identify areas for excavation/removal. Remaining contamination can then be addressed by subsurface remedies that will achieve concentrations below vapor intrusion concentrations of concern. Bulk sampling is unreliable for predicting whether VOC concentrations detected in the soil or groundwater will migrate as vapor into structures (EPA, 2014).
↩Sub-Slab Sampling
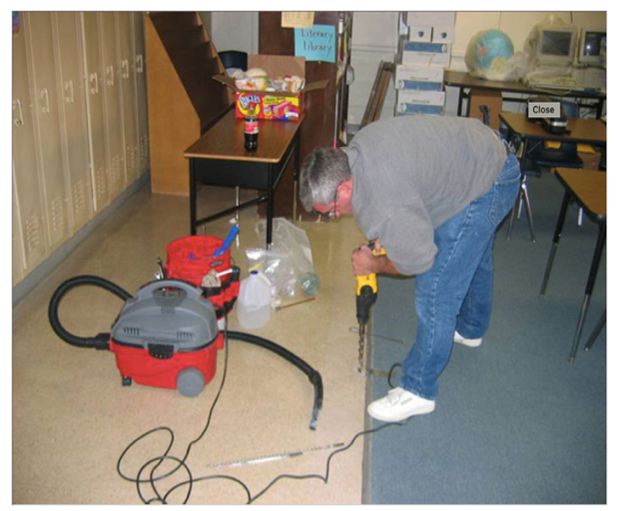
Figure 4. Advancing a Sub-Slab Soil Gas Probe (U.S. EPA Region 5, 2020).
In addition to collecting soil gas data from a potential vapor intrusion source, the investigator may want to assess the soil vapor directly beneath a building where there is a concern about indoor air quality. This is done by collecting sub-slab soil vapor samples. Sub-slab sampling is the collection of a soil vapor sample from beneath a building's slab foundation. Sub-slab sampling is typically employed when the CSM and existing data (e.g., groundwater or soil vapor) suggest that vapor intrusion through the foundation may be a concern. A sub-slab soil gas sample is obtained by coring or drilling through the slab to insert a probe (Figure 4). Placement of the probe is targeted to air spaces that have formed beneath the concrete over time or the pore space within the granular material placed below the slab during construction. Sub-slab data may be useful in determining whether the subsurface vapor migration pathway is complete and poses a potential health concern. Additional considerations regarding sub-slab soil gas sampling can be found in EPA's 2015 Vapor Intrusion Technical Guidance.
↩Conduit/Utilities Sampling
As part of the vapor intrusion investigation, the site should be evaluated to identify the presence of utilities or conduits that may facilitate vapor transport across the site and potentially into buildings or structures that are serviced by the utilities. A search of public and facility records for as-built diagrams, construction specifications and/or locations of the utilities may be warranted (EPA, 2015).
As in the ESTCP Project ER-201505 report, the following sampling methods may be used to assess the contribution to vapor intrusion in buildings/structures via conduits and utility tunnels:
- Collect vapor samples from sewer or conduit - If the conduit or sewer has liquid in it, collect a vapor sample by lowering the collection device to within 1 foot of the bottom or the liquid in the conduit, whichever is shallower. Connect a small-diameter nylon tubing to a three-way valve to allow purging of the line and sample collection. After at least three line volumes of vapor are purged, a sample container can be attached for collection of a sample.
- Collect liquid samples from sewer or conduit - Liquid samples should be collected in the appropriate VOA vials provided by the analytical lab. The same sample protocols for handling groundwater samples apply to liquid samples from the sewer or conduit.
- Tracer Testing - Research conducted by McHugh, T., et al. (2017) at the EPA vapor intrusion research duplex in Indianapolis, Indiana, demonstrated that tracer testing could be used as a tool to determine whether conduits such as sewer lines are contributing to vapor intrusion into a structure.
Tracers such as perfluorocarbon4 tracers (PFTs) are deployed within the sewer or conduit as sources. Each source emits a different perfluorocarbon compound. Passive samplers are stationed within various locations of the structure to collect data for assessing if and when a particular perfluorocarbon compound (tracer) reaches the sampler. In this way, the vapor intrusion contribution can be "mapped" from different sources such as sewer lines, utility conduits, etc. Data from the tracer test, used in conjunction with sewer liquid and vapor samples, sub-slab vapor samples, indoor air samples and soil gas samples can determine whether sewer lines or other utilities act as conduits for intrusion of contaminant vapors into structures.
↩Air Sampling
Air samples are collected to assess whether vapors are present in the building at levels that pose a health risk. Indoor air data can also be used to evaluate the performance of vapor mitigation systems. Interpreting indoor air sampling results can be complicated by indoor and outdoor air sources of VOCs unrelated to the subsurface contaminant source (see EPA, 2015 and EPA, 2011).
Sample collection methods include pressurized (active) and unpressurized (passive) evacuated canisters and active and passive sorbent samplers. Canisters provide the benefit of "whole air" samples (i.e., collection of the vapor phase itself), allowing multiple sub-samples and the ability to analyze for a wide variety of compounds. The canisters are typically left in place for a period of 8 hours in commercial settings and 24 hours in residences (EPA, 2015). Passive sorbent samplers provide the ability to collect time-integrated samples over longer periods, as recommended in EPA's Vapor Intrusion Technical Guidance, in part to reduce the likelihood of obtaining false-negative results (Nocetti, D., et al., 2019). Disadvantages of sorbent samplers include the need to pair the sorbent material with the chemicals of interest, and potentially being limited to only one analysis for each individual device. Additional information regarding indoor air sampling can be found in EPA's Vapor Intrusion Technical Guidance.
↩Analytical Methods
Analytical methods used in vapor intrusion site assessment should be capable of achieving detection limits below applicable screening criteria such as the levels identified by EPA's Vapor Intrusion Screening Level Calculator. The calculator provides general risk-based target concentrations for groundwater, near source soil gas and sub-slab soil gas, and indoor air. Table G-5 of ITRC's 2014 Petroleum Vapor Intrusion guide provides a list of parameters and the appropriate associated analytical methods.
↩
Building design will impact the potential for vapor intrusion to be a complete pathway for human exposure and should be considered in developing and refining the CSM. Appendix C of ITRC's 2007 Vapor Intrusion Pathway: A Practical Guide provides a more detailed discussion of the various factors in building design that can affect the potential for vapor intrusion into buildings. These factors include but are not limited to:
- Building air exchange.
- The presence or absence of cracks, seams, gaps in basement floors, walls, or foundations.
- The presence or absence of openings for utilities in basement floors, walls, or foundations.
- Whether the building is constructed with a crawl space, basement or is built on a slab on grade.
In addition to building factors, recent research has shown that sanitary sewers, land drains, and utility tunnels can act as preferential pathways for vapor intrusion (e.g., Guo et al 2015, McHugh and Beckley 2018). Sites are typically at higher risk for sewer vapor intrusion if the sewer lines directly intersect subsurface VOC sources. Thus, sewer preferential pathways should be considered during development of the CSM and the investigation program.
Vapor intrusion investigation approaches continue to be developed. Ma et al (2020) summarizes a variety of conventional and innovative methods currently in use. These methods include the following:
High Purge Volume Sub-slab Sampling/Pneumatic Conductivity Testing
Sub-slab soil gas sampling is a common line of evidence in vapor intrusion investigations. However, spatial variability is common and can increase the risk of failing to identify elevated chemical concentrations beneath the building. To overcome this problem, high purge volume sampling can be done to spatially average the sub-slab concentrations and obtain an improved understanding of conditions beneath a given building(McAlary, et al., 2010).
This method can also be applied to support design of mitigation systems. It is analogous to pneumatic testing for soil vapor extraction system pilot tests. Application of suction and measuring the vacuum can be used for pneumatic conductivity testing of subsurface geologic layers. Data from pneumatic testing may be used to determine whether a laterally continuous soil layer acts as a barrier to upward soil vapor transport, or of a building's floor slab to optimize the design of sub-slab depressurization or venting systems.
Meteorological Monitoring
Weather conditions can influence both soil gas and indoor air concentrations of contaminants. Meteorological parameters that may affect soil gas and indoor air concentrations include (ITRC, 2007):
- Rainfall events - Vapor intrusion rates and concentrations of soil gas can be affected by precipitation. Significant rainfall events may skew data collected during or immediately after the event and, therefore, may not be representative of long-term conditions.
- High wind speed - Pressure differentials can be created around a structure during high wind events. This can cause an advective flow in shallow soil gas around and beneath the structure.
- Frozen ground or permafrost - When the ground is frozen, the flow of air into the vadose zone and/or the flow of soil gas out of the vadose zone may be restricted.
- Major storm events - A process known as barometric pumping can occur due to changes in barometric pressure creating movement in the near surface vadose zone.
Because weather can affect soil gas and indoor air concentrations, meteorological monitoring (and monitoring of building differential pressure) during sample collection can provide another line of evidence for interpretation of vapor intrusion investigation data.
Forensic Approaches
Because indoor sources of VOCs are ubiquitous, it can be difficult to distinguish whether VOCs found in indoor air samples are from indoor sources or the subsurface (i.e., vapor intrusion). Several innovative methods are now available to help differentiate the chemical profiles of subsurface sources (i.e., groundwater, soil gas) and indoor air samples. Forensic approaches include hydrocarbon fingerprinting and forensic analysis, CSIA (compound-specific isotope analysis), on-site chemical analysis, and the use of radon as a tracer (NAVFAC, 2013; Ma et al 2020).
Guo, Y., et al., 2015. Identification of Alternative Vapor Intrusion Pathways Using Controlled Pressure Testing, Soil Gas Monitoring, and Screening Model Calculations. Environmental Science & Technology, 49:22, p. 13472-13482, October 12.
Interstate Technology Regulatory Council (ITRC), 2014. Petroleum Vapor Intrusion: Fundamentals of Screening, Investigation, and Management. 388 pp, October.
Interstate Technology Regulatory Council (ITRC), 2007. Vapor Intrusion Pathway: A Practical Guideline. 172 pp, January.
Ma, J., et al., 2020. Vapor Intrusion Investigations and Decision-Making: A Critical Review. Environmental Science & Technology, 54:12, p. 7050-7069, May 8.
McAlary, T., et al., 2010. High Purge Volume Sampling-A New Paradigm for Subslab Soil Gas Monitoring. Groundwater Monitoring & Remediation, 30:2, p. 73-85, May 12.
McHugh, T. et al., 2017. Evidence of a Sewer Vapor Transport Pathway at the USEPA Vapor Intrusion Research Duplex. BLN-113837-2017-JA. 18 pp, April.
McHugh, T. and L. Beckley, 2018. Sewers and Utility Tunnels as Preferential Pathways for Volatile Organic Compound Migration into Buildings: Risk Factors and Investigation Protocol. ESTCP Project ER-201505. 791 pp, November.
Naval Facilities Engineering Command (NAVFAC), 2013. Innovative Vapor Intrusion Site Characterization Methods. 8 pp, February.
Nocetti, D., et al., 2019. Sampling Strategies in the Assessment of Long-term Exposures to Toxic Substances in Air. Remediation Journal, 30:1, p. 5-13, December.
U.S. EPA Region V, 2020. Vapor Intrusion Handbook. Superfund and Emergency Management Division. 150 pp, March.
U.S. EPA, 2015. OSWER Technical Guide for Assessing and Mitigating the Vapor Intrusion Pathway from Subsurface Vapor Sources to Indoor Air. OSWER Publication 9200.2-154. 267 pp, June.
U.S. EPA, 2014. Challenges in Bulk Soil Sampling and Analysis for Vapor Intrusion Screening of Soil. Office of Research and Development. EPA/600/R-14-277. 14 pp, December.
U.S. EPA, 2011. Background Indoor Air Concentrations of Volatile Organic Compounds in North American Residences (1990-2005): A Compilation of Statistics for Assessing Vapor Intrusion. EPA 530-R-10-001. EPA, 67 pp, June 2011.
Helpful Definitions
Conceptual site model (CSM): A CSM is a picture and narrative of the site contamination: how it got there, whether or not it is migrating or degrading, its distribution across the site, who might be exposed to it, and what risk-reduction strategies are most feasible. ↩
Conceptual site model (CSM): A CSM is a picture and narrative of the site contamination: how it got there, whether or not it is migrating or degrading, its distribution across the site, who might be exposed to it, and what risk-reduction strategies are most feasible. ↩
Active Gas Soil Sampling: Active soil gas sampling involves the collection of soil gas by pumping a volume of soil gas from the target zone. Samples can be analyzed immediately. Active soil gas sampling facilitates rapid assessment/expedited characterization if volatile organic compounds are primary constituents of concern for vapor intrusion. Soil gas samples can be collected with temporary or permanent sampling probes. Probes can be installed either using augered soil borings or a direct push method such as the Geoprobe�. The sampling tubing typically has a small diameter (<1/4 inch inside diameter) and made of copper, stainless steel or nylon. The tubing runs from the ground surface to the target depth. Sampling can be done at a single target depth or done in clusters where more than one sampling probe is installed at several target depths to define a vertical profile of soil gas concentrations (EPA, 2015 and EPA Region 5, 2020). ↩
Active Gas Soil Sampling: Active soil gas sampling involves the collection of soil gas by pumping a volume of soil gas from the target zone. Samples can be analyzed immediately. Active soil gas sampling facilitates rapid assessment/expedited characterization if volatile organic compounds are primary constituents of concern for vapor intrusion. Soil gas samples can be collected with temporary or permanent sampling probes. Probes can be installed either using augered soil borings or a direct push method such as the Geoprobe�. The sampling tubing typically has a small diameter (<1/4 inch inside diameter) and made of copper, stainless steel or nylon. The tubing runs from the ground surface to the target depth. Sampling can be done at a single target depth or done in clusters where more than one sampling probe is installed at several target depths to define a vertical profile of soil gas concentrations (EPA, 2015 and EPA Region 5, 2020). ↩
Passive Gas Soil Sampling: Passive soil gas surveys deploy absorbent materials in the ground and left for days or weeks. Contaminant vapors are collected on the absorbent material via the ambient flow of soil gas. An advantage to passive sampling is that the samplers can be placed in locations where power is unavailable and can be left unattended for long periods of time (EPA, 2015 and EPA Region 5, 2020). ↩
Passive Gas Soil Sampling: Passive soil gas surveys deploy absorbent materials in the ground and left for days or weeks. Contaminant vapors are collected on the absorbent material via the ambient flow of soil gas. An advantage to passive sampling is that the samplers can be placed in locations where power is unavailable and can be left unattended for long periods of time (EPA, 2015 and EPA Region 5, 2020). ↩
Perfluorocarbons (PFCs): Perfluorocarbons (PFCs) are compounds found at low levels in the atmosphere and present no identified danger to humans if inhaled or ingested. They are chemically inactive, nontoxic and nonflammable (Brookhaven National Laboratory). ↩
Perfluorocarbons (PFCs): Perfluorocarbons (PFCs) are compounds found at low levels in the atmosphere and present no identified danger to humans if inhaled or ingested. They are chemically inactive, nontoxic and nonflammable (Brookhaven National Laboratory). ↩